How to Measure a Spectrum
Breaking Light into a Spectrum: Dispersion and Diffraction
Just about every astronomy textbook you will ever pick up will contain a phrase to
the effect that the process of breaking light up into a spectrum is "like passing
white light through a prism." This process, called dispersion, arises
because different colors (or wavelengths) of light bend by different amounts
as they pass from, say, a low density medium (like air) into a higher density medium
(like the glass in a prism). Hence, a narrow beam of "white" light will
get spread out into a rainbow. Voila, a spectrum!
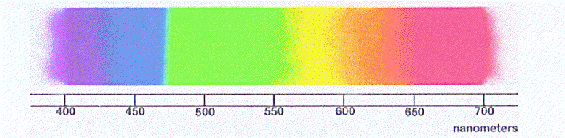
The colors of the familiar "rainbow" of visible light correspond to differing
wavelengths of the light, here shown on a nanometer scale. The wavelengths get
successively larger as one moves from left to right.
But such a spectrum, although very pretty, is of very little use to astronomers.
This kind of spectrum does not convey the detailed physical information that we require
to do science. And as a practical matter, some kinds of light (such as ultraviolet
light for example) do not pass through a glass prism but rather are absorbed! It is
tough to measure a spectrum when the light gets absorbed!
In practice, most spectrographs in astronomy, including those that operate in the
optical part of the spectrum, use a totally different method for creating a spectrum
out of the incoming light from the telescope--the process of
diffraction. This process depends on the wave-like properties of the light,
and uses a component called a diffraction grating to actually separate the
light into its component wavelengths. A diffraction grating
consists of a substrate (often made of glass, but stainless steel, plastic, or other
materials are sometimes used) onto which are etched very narrowly-spaced lines.
How narrowly-spaced? Well, a typical diffraction grating used in optical astronomy
may have anywhere from several hundred to over one thousand lines etched
per millimeter! A famous physicist from Johns Hopkins University,
Henry A. Rowland, was the
first person to make high quality diffraction gratings for use in scinece.
How does such a grating break a beam of light into its component wavelengths?
[TBD. Hope to add this soon! But most Intro Physics text books give a description
of this process. So until I get around to it, use the Library!]
Spectroscopes and Spectrographs
A diffraction grating by itself is really no better than a prism for creating an
astronomical spectrum. The grating must be built into a device called a
spectroscope or spectrograph for this to be done. These are
effectively the same thing except that a spectroscope
is simply used for visual inspection (that is, your eye is the detector), while a
spectrograph includes some means (photographic film or an electronic detector) for
recording the spectrum for analysis. In professional astronomy these days there is very
little need for a spectroscope (just as there is very little other observational
work actually done with the naked eye, with the possible exception of staring at
a computer monitor all day!).
Ok, so what is a spectrograph? In its simplest form, it is a light-tight box with
a small (often narrow rectangular or adjustable) opening to let light in, a grating
to break the light into its components, and a "detector" of some kind placed at the
proper angle and distance from the grating to record the spectrum of the wavelength
range of interest. Telescopes are used to gather the faint light from distant
objects, and the spectrographs are placed at the focus of the telescope to analyze
the light.
Detecting and Recording Spectra
A detector is simply a device that senses and measures the incoming light. In
a spectrograph, the detector has to perform this task across a range of wavelengths,
measuring the amount of light as it changes from wavelength to wavelength. In an
optical spectroscope, the detector is your eye, which senses the different colors
and the presence of dark absorption lines or bright emission lines in the spectrum
of the source being viewed. In a spectrograph, some other device is used to sense
the light.
For many years the primary detector used in spectrographs was the photographic plate
(basically film, although special astronomical emulsions placed on glass plates
were used for greater sensitivity and stability). Often spectra recorded this way
were then traced with a device called a (are you ready for this?)
microdensitometer. This device would shine a steady, narrow beam
of light through the photographic plate to a light sensitive photomultiplier tube.
As the plate was stepped along the length of the spectrum, the photomultiplier
tube would measure and record the amount of light at each wavelength.
The resulting tracing would essentially be a graph of the
intensity of light as a function of the position on the photographic plate (or as
a function of wavelength in the case of a spectrum). This graphical representation
of a spectrum is what astronomers find most useful in doing their work.
This picture shows an electronic detector called a charge-coupled device, or CCD. The small
central rectangle contains a closely packed array of 320 by 512 light sensing diodes, each
of which individually record the brightness of light and send the information to a computer.
Imagine placing this device at the focus of a large telescope! It allows astronomers to "see"
objects millions of times fainter than the unaided eye! (Click on the picture to
see a larger version. Photo courtesy of the Smithsonian Astrophysical Observatory.)
Over the last 20 years or so even photographic recording of spectra has nearly become
a thing of the past. Electronic recording of spectra is the most sensitive,
quantitative, way of detecting the light, and it gets the spectrum directly into
a digital form that can be handled on a computer (where the real work gets done).
The detector used most often in astronomy these days is called a charge coupled
device, or CCD. This device is basically an array of tiny, light-sensitive
diodes and is
also now commonly used in video cameras and digital still cameras. Astronomical
CCDs, however, are often tweaked
up to provide the best performance at faint light levels, in many cases recording
the arrival of individual photons of light from distant sources in the Universe!
Resolving Power versus Spectral Coverage
Every time an astronomer goes to a telescope to obtain spectra, he/she has to answer
several questions about the goals needed for their investigation. For instance,
one has to know exactly what spectral lines need to be observed, and hence, how
much spectral coverage is necessary. Are all of the lines of interest in
the red part of the spectrum, or is full spectral coverage from the blue through
the red needed? The other basic question is how much resolving power
is needed (basically, how much does the light need to be spread out to show the details
in the spectrum)?
This last question involves several considerations. Are there spectral lines of interest
that are close together in wavelength? If so, one must use sufficiently high dispersion
to allow the lines to be separated; otherwise the lines will be blended together such
that they cannot be measured individually.
Another consideration may be whether one is making velocity measurements. If so,
what precision is needed for measuring the redshifts or blueshifts of the lines in
the spectrum? For instance, let's say you wanted to measure velocities of expansion
of a planetary nebula, which are typically about 10 kilometers per second, using lines in
the red part of the optical spectrum (about 6500 Angstroms). The equation for Doppler
shifts says you would want to make sure your spectrograph can make measurements to
an accuracy of at least 0.2 Angstroms.
A difficulty sometimes arises when a project desires both high spectral dispersion
and broad wavelength coverage. For a detector of fixed size, the more one spreads
the light out (higher dispersion) the less the range of wavelengths that will fall
on the detector (smaller spectral coverage). In cases where both spectral coverage
and high spectral dispersion are needed, a special spectrograph called an
echelle spectrograph can be used. This device contains two diffration
gratings instead of one, a high
dispersion grating to provide the desired spectral resolution, and a lower dispersion
grating that spreads the overall spectrum out into an array of miniature spectra,
each covering only a portion of the desired spectral range. While these
spectrographs are not suitable for every observation, they make it possible in
certain instances for a single observation
to do the job of 50 or more observations with a regular spectrograph!
Click here to go to next section.
Return to Spectroscopy Home Page.
Bill Blair ([email protected])